Introduction to NGS
Next gene sequencing is a technology that identifies the DNA or RNA sequence in order to explore genetic variation linked to diseases or other biological phenomena. This technique was first made available for commercial use in 2005. It was first called “massively-parallel sequencing” because it allowed the sequencing of several DNA strands at the same time instead of one at a time like traditional Sanger sequencing by capillary electrophoresis (CE).
In the context of genetic analysis today, each of these tools is useful. Sanger sequencing can be completed in a single day and is excellent for examining modest quantities of gene targets and samples. Sanger sequencing is likewise regarded as the industry-standard sequencing technology, so NGS results are frequently validated using it. A single sequencing run of NGS allows for the discovery and analysis of a variety of genomic features, including single nucleotide variants (SNVs), copy number and structural variants, and even RNA fusions.
NGS also allows for the simultaneous analysis of hundreds to thousands of genes across multiple samples. NGS offers the best throughput per run, enabling speedy and economical study execution. NGS is also better than Sanger sequencing because it needs less sample input, is more accurate, and can find mutations at lower allele frequencies.
What is the process of next-generation sequencing?
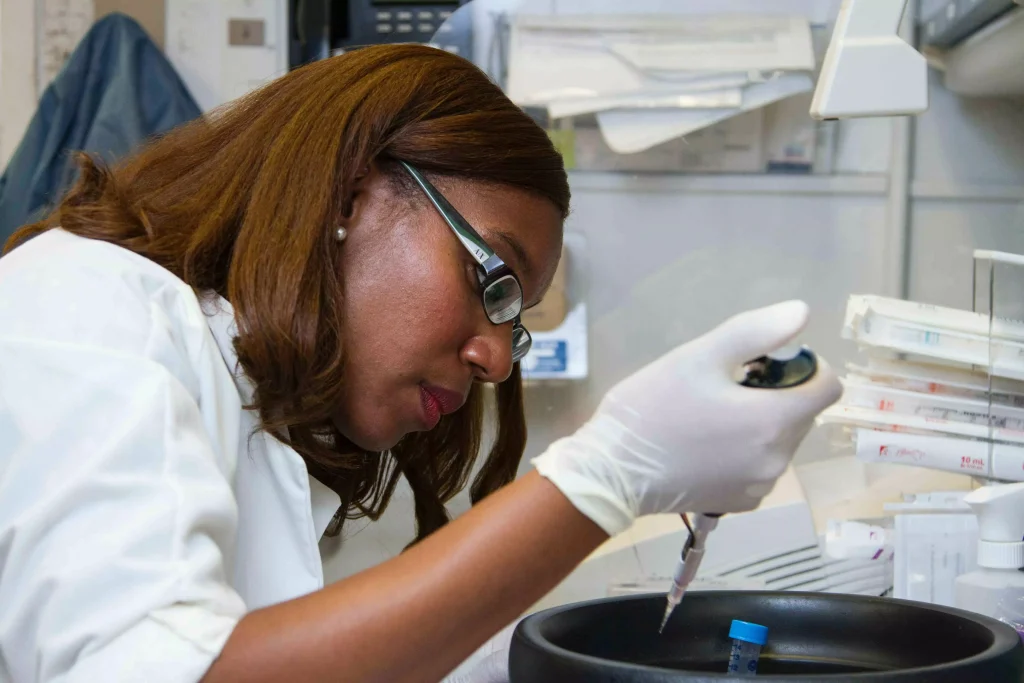
Next-generation sequencing technologies primarily work by combining short reads from fragmented DNA through step-by-step cycles of polymerase-based extension or oligonucleotide ligation. But the newest methods for next-generation sequencing can make longer reads from much longer segments of DNA nucleotide strands.
There are two tried-and-true methods for delivering long-read sequencing. In the first method, known as nanopore sequencing, the strands are passed through a protein nanopore while electrical current changes are recorded for each base.
The sequence is then decoded by a computer using these modifications. In the second method, known as single-molecule real-time (SMRT) sequencing, the strands are circularized and tagged bases that emit light upon integration are incorporated using a polymerase. Real-time measurements of nucleotide incorporation are made using the light that is detected.
New development frontiers are broken in next-generation sequencing technologies on an almost annual basis. The most recent advancements incorporate single-cell sequencing, which avoids ensemble average readings from a sample, which may be deceptive, and provides more accurate insight into the nucleic acids of specific cells during a given phase or time point.
Spatial sequencing is a new way to directly sequence from tissue or sample. This gives researchers a better idea of how the cell works in its natural environment and how its parts are put together.
The development of next-generation sequencing
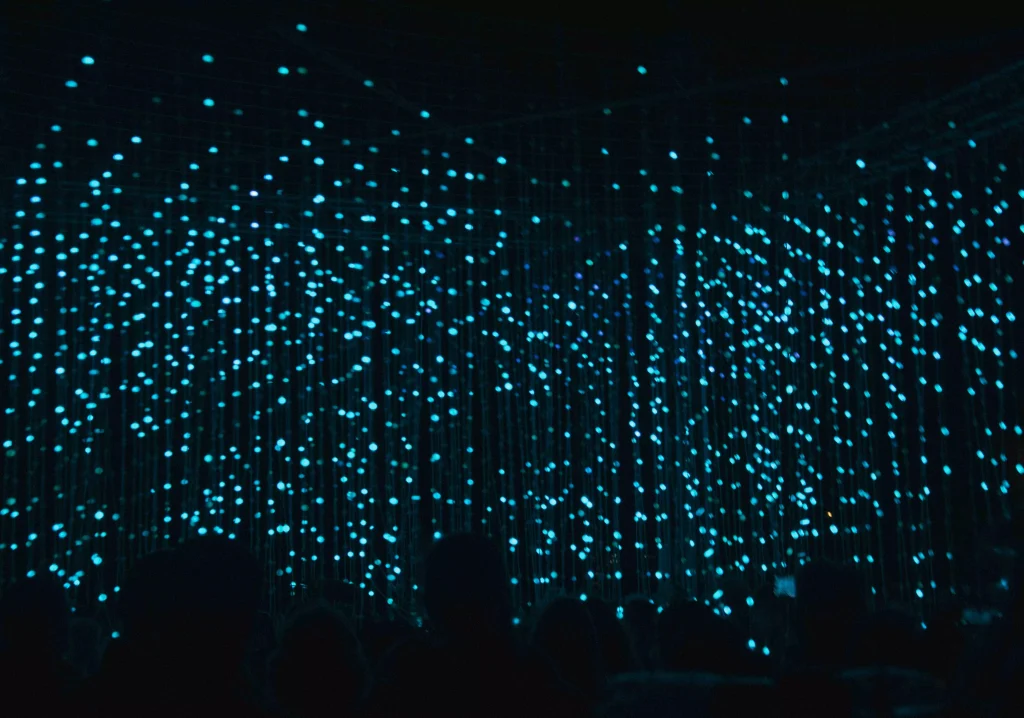
Following the conclusion of the Human Genome Project in 2003, these methodologies have been developed throughout the course of the subsequent two decades. During this time period, Sanger sequencing was utilized to assemble the fragments of the human genome in a project that lasted for 13 years and cost approximately $2.7 billion.
Since then, the speed of sequencing has significantly improved, while at the same time, prices have significantly decreased. Since 2018, the price of sequencing an entire human genome has fallen below $1000, and the process of sequencing an entire human genome currently takes less than a day to complete.
Uses and applications of NGS
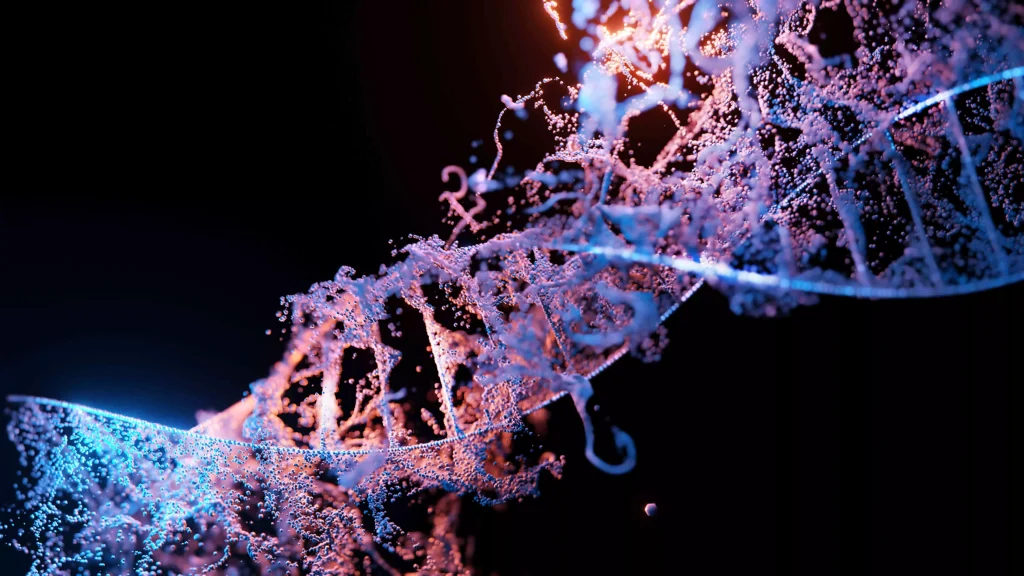
NGS offers a wide range of uses, which enables quick technological development in numerous branches of biological science. The human genome is currently being re-sequenced in order to find genetic elements that influence disease. Researchers have been able to learn a great deal about a variety of organisms thanks to NGS and whole-genome sequencing.
In epidemiology and public health research, the technology is also used to sequence bacteria and viruses to find potential virulence-related variables in bacteria and viruses. Additionally, NGS of RNA has begun to displace microarray analysis in gene expression studies, allowing researchers to view the sequence of RNA expression.
This RNA sequencing can give information about an organism’s whole transcriptome in a single analysis, even if you don’t know its genetic sequence. These are only a few ways that NGS can help researchers and medical professionals, and as the technology is employed more frequently, further uses for it will certainly be created.
Standard sequencing applications
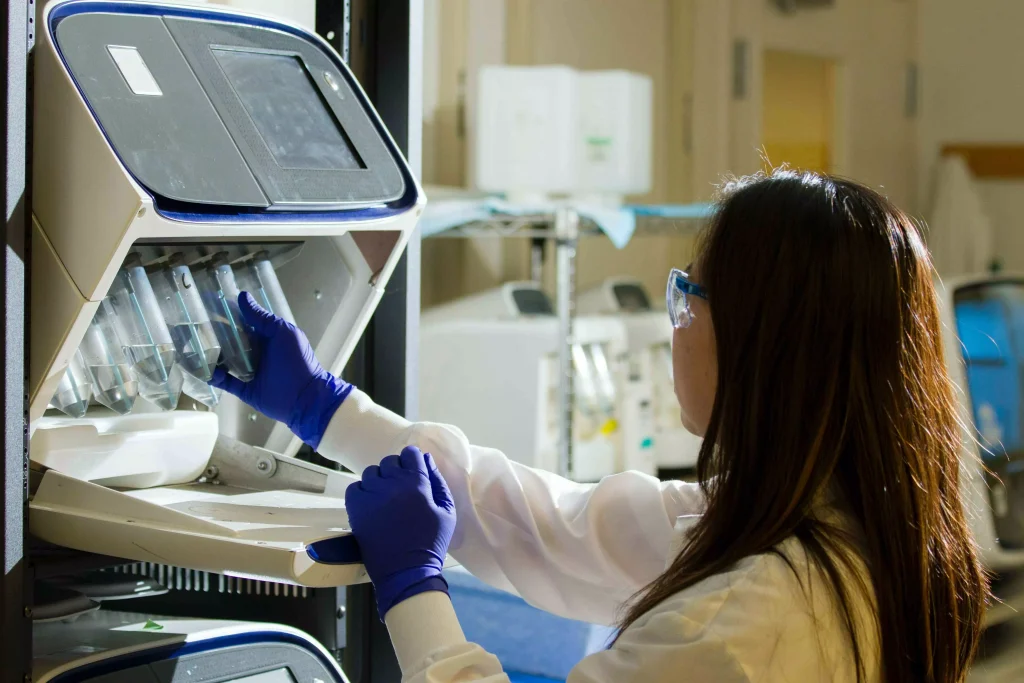
Research on cancer: Cancer researchers can find unusual somatic variations, tumor subclones, and circulating DNA fragments using NGS-based sequencing techniques.
In microbiology research, sequencing can help scientists learn about the genes of bacteria and viruses. This can help them with a wide range of studies, such as environmental metagenomics analyses, infectious disease surveillance, and more.
Research on complex diseases: Understanding autoimmune and rheumatic diseases, atherosclerosis, neurological diseases, and psychiatric problems at the molecular level is becoming possible thanks to Illumina sequencing.
Reproductive Health: Illumina’s sequencing and an array of technologies give fast and accurate information that can help people make decisions that will improve their reproductive health.
Positive aspects of NGS
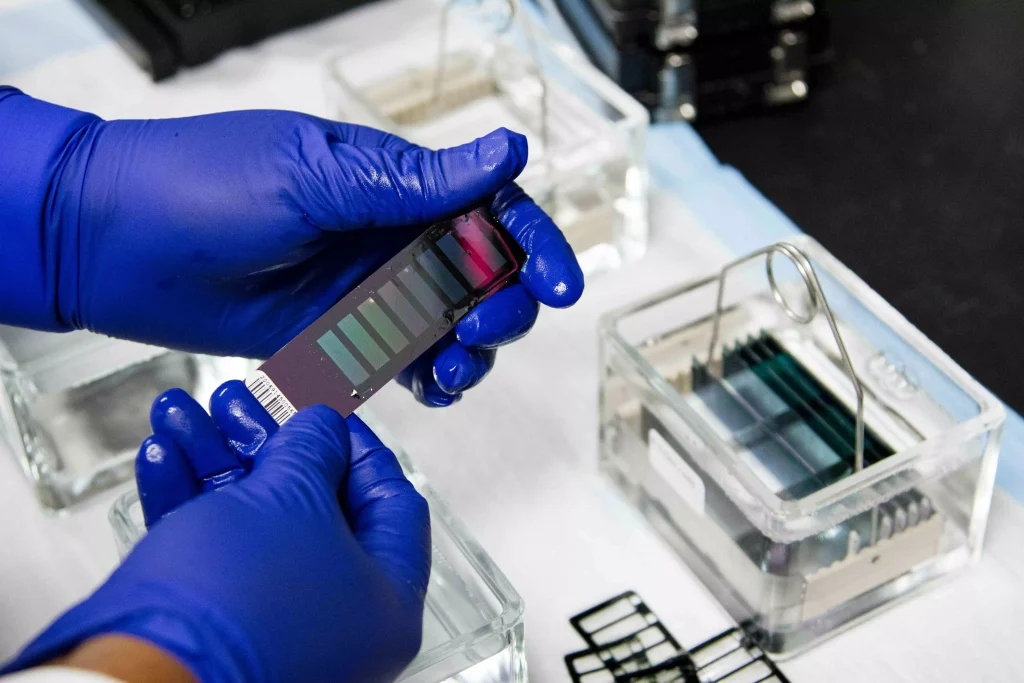
NGS is a prominent method in the field of functional genomics because it can be used to analyze samples of both DNA and RNA. In contrast to methods based on microarrays, NGS-based approaches provide a number of advantages, including the following:
- It is not necessary to have prior knowledge of the genome or of the features of the genome.
- It provides single-nucleotide resolution, which makes it feasible to find related genes (or features), alternatively spliced transcripts, allelic gene variants, and single nucleotide polymorphisms;
- a greater dynamic range for the signal.
- It needs less DNA/RNA as an input (nanograms of materials are sufficient).
has a greater capacity for reproducibility.
NGS boundaries
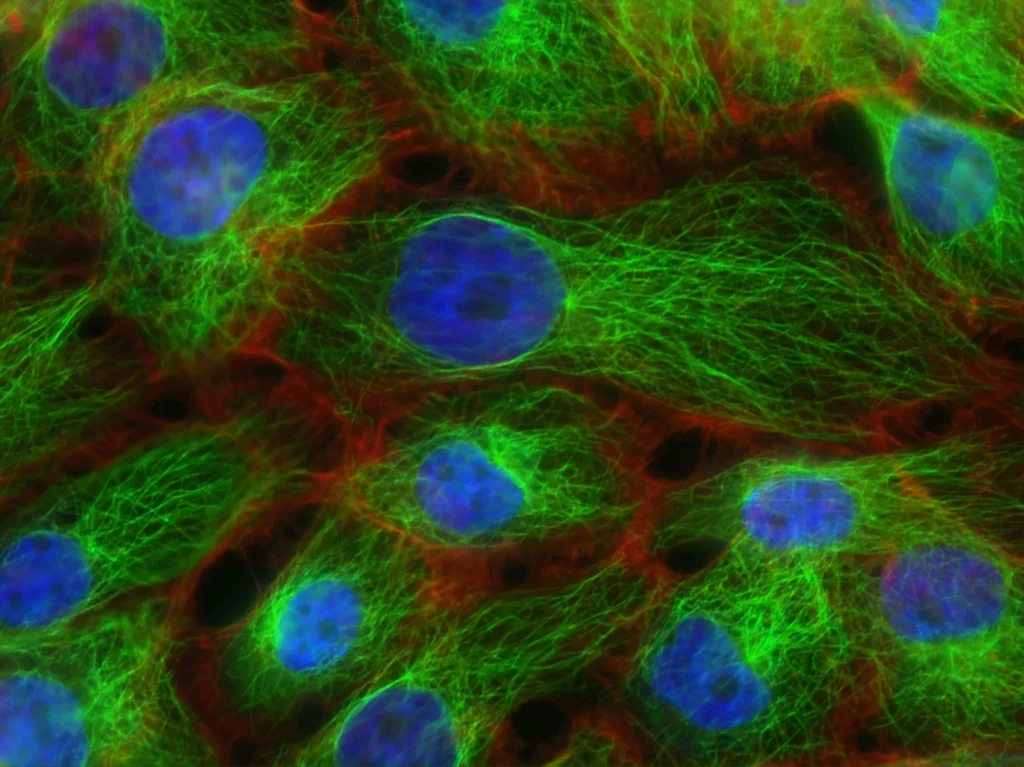
The biggest drawback of NGS in a clinical environment is the need to build up the necessary infrastructure, such as computer power and storage, as well as the staff know-how needed to thoroughly analyze and interpret the resulting data.
The volume of data must also be skillfully managed in order to extract clinically significant information in a transparent and reliable interface. The actual cost of NGS sequencing is very low. For instance, a cutting-edge NGS platform can produce roughly 150 000 000 reads for about £1000, compared to the typical cost of a single Sanger read, which is less than £1.
To make NGS cost-effective, massive batches of samples would need to be run, which might call for supra-regional centralization. The ability of an NGS facility to provide a service on a national scale after the first capital investment is made is expected to have economic benefits in addition to advances in patient care.
Clinical conclusion
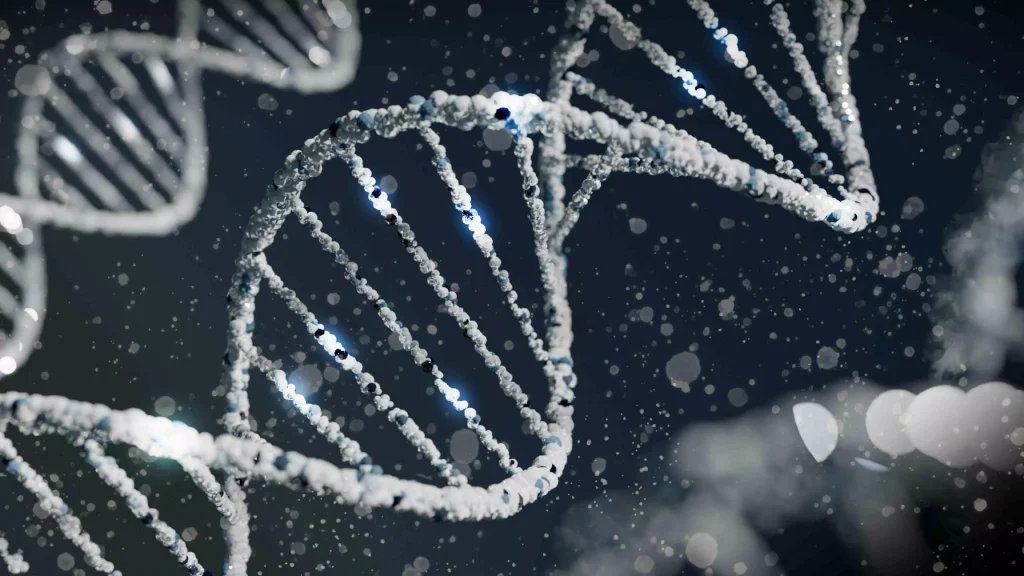
- Although NGS has a lot of potentials, research is currently its main application.
- NGS will enable pediatricians to take genetic data to the patient’s bedside.
Read more articles in the Technology Category